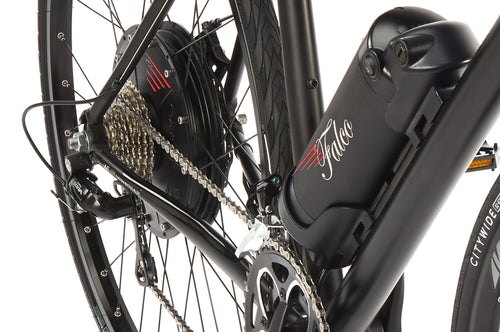
Review of eBike Motor Technologies

Review of eBike Motor Technologies
Electric bikes and light electric vehicles of less than 1000 Watts are the only commercially viable mode of alternative transportation in the market today. Electric or fuel-cell cars are not fully commercially viable and will not be viable for a long time. Europe, Asia and many US cities have adapted to electric bike transportation easily and skillfully. A pedal-assist-electric-bike can easily deliver a range of 100 km+ with a 36V, 11.6Ah Li-Ion battery.
This paper reviews and compares Falco’s highly-efficient and light weight direct drive electric propulsion technology (DDEP) for light electric bicycles against competitive technologies. Light electric vehicles provide the greatest potential of being able to reduce greenhouse emissions and our dependence on fossil fuels. An electric bike ride emits 15.8g CO2 per mile as compared to 368g CO2 per mile for a mid-sized passenger car. These vehicles have seen most growth in the last two years in Western Europe, Canada, Asia and the United States. Electric two-wheeler market in Western Europe and North America is in excess of one million vehicles per year and growing at 17% annually. However, there are five major impediments to market growth –poor portability, limited range, pedaling difficulty, low top speed and low hill climbing ability. With Falco's eBike system, it may be possible to diminish these factors significantly. Falco’s technology provides an electric propulsion technology with at least 25% better portability (i.e., lower weight), 10% more range (i.e., more efficiency), 25% reduction in pedaling difficulty (i.e., cogging torque), 25% more top speed (i.e., more power) and higher hill climbing ability (i.e., more torque) than existing systems. This paper reviews key propulsion components such as motor design, motor inverter architecture, motor control and energy management methods for light electric vehicle application.
While the three-phase motor architecture, fractional slot concentric winding (FSCW) configuration, radial geometry and trapezoidal or sinusoidal control methods have been the back-bone of existing DDEP systems in light electric vehicles, Falco’s five-phase technology has made it possible to employ an innovation in the motor architecture, winding configuration, control and energy management methods to improve the system significantly.
The enabling research innovation presented here makes use of a novel poly-phase multi-pole outer rotor radial flux motor architecture. Falco’s magnetic circuit arrangement minimizes laminations use, resulting in the reduction of motor volume and weight. A unique numbers of poles and slots are used such that the magnetic force between the stator and the rotor at any rotating position is uniform, hence eliminating the cogging torque. A novel hybrid winding configuration with reduced copper weight ensures high power density, torque density and system efficiency resulting in more range. The inverter is integrated into the interior of the motor to improve efficiency, reduce cost and save space. Control methods use low cost torque and speed control with minimum battery consumption and smooth acceleration from stand-still.
Falco’s innovation possesses the distinct advantages of low weight, high efficiency, low cogging, high power, high torque, and superior dynamic performance and could result in accelerated acceptance of Light Electric Vehicles as a serious mode of transportation.
Such a technology has never been explored before in the field of motor design and control due to lack of need, perceived complexity and higher costs in R & D activities and manufacturing. However, with ever decreasing costs of hardware implementation and a pressing need for a highly efficient and light weight DDEP technology, it has now been feasible to develop such a system.
FALCO’s five-phase technology addresses the highlighted needs for vehicle weight reduction and improved efficiency, fuel processing technologies, alternative fuel options and SOx and NOx reduction through a focus on a direct drive electric propulsion technology. FALCO’s particular emphasis has been on the design and development of such a system with control methods required for extra low weight and high performance.
Review of State of the Art
Permanent Magnet Motor Technology
Permanent magnet (PM) motors have synchronous machines are preferable choice in light electric vehicles (LEVs) due to low weight and volume, high torque and power density, excellent controllability and reliability, simplicity and ruggedness, high efficiency and low maintenance. They have superior advantages in variable speed and load applications compared with induction, switched- and variable-reluctance motors [1, 34]. In recent years, poly-phase PM motors of 3-, 4-, 5-, 6- and 7-phase configuration have been investigated [1, 6, 7, 8, 9, 10, & 11]. Five-phase motors have shown promise to improve performance over three-phase configurations.
Research has also been carried out in radial, axial, transverse and Halbach array flux architectures [17, 18, 19, 20, 21, & 22]. The radial flux motors (RFM) has been widely used in the industry due to relative ease in manufacturing. Axial flux motors (AFM) have been difficult to manufacture due to the high expense of fabricating lamination stacks. Axial flux motors have shown superior performance over radial flux motors where length of the motor is less than 30% of the diameter (length/diameter<0.3) [17]. Transverse flux motors (TFM) are more difficult to design and manufacture. Halbach array motors have shown promise for operations requiring significantly higher speed and are not considered suitable for low speed, high torque operations [21, 22].
The current state of the art for permanent magnet propulsion motors is depicted in Figure 2. Essentially, Permanent magnet motors can be configured to have an outer rotor (i.e., inverted) or inner rotor (i.e., non-inverted) configuration. A motor can also have a magnet arrangement to form radial, axial, transverse or halbach flux topology. Number of poles or slots and number of motor phases are decided by required torque, power, speed, weight, dimensions, torque ripple, fault tolerance etc specifications. The selection of materials is largely dictated by volume, weight and cost requirements.
In the sub-sections below, we briefly discuss some key concepts influencing the design of DDEP systems for LEVs.
Gearless Hub Motors:
Gearless Hub motors have the unique advantage of transferring torque and power directly to the road [23]. Such is not the case with geared hub motors or current automotive drive trains. There is a significant loss of efficiency in belt or chain driven systems with gear drives. Low speed high torque gearless hub motors used for propulsion application are not as efficient as high speed motors. Nevertheless, the overall propulsion system efficiency is much higher with gearless hub motors [24]. Automotive companies have avoided hub motors due to concerns related to vehicle dynamics. Schalkwyk et al [25] in their research observed that added wheel mass has no effect on the stability of a vehicle and that the frequency response is within the accepted comfort range. Chen et al [26] presented a study on the use of two rear hub motors and concluded that yaw rate and trajectory are improved when steering input is applied. Kazmin et al [27] built, designed and tested an 80-kW surface-mounted PM (SPM) in-wheel hub motor for hybrid truck.
Five Phase Motor Architecture:
Dr. Leila Parsa of Rensselaer Polytechnic Institute and Dr. Hamid Toliyat of Texas A&M University are leading researchers in this field [9]. Five phase motors have unique characteristics which are not yet commercially exploited. Five phase induction motor drives have demonstrated significant lower torque ripple, higher power density, higher torque density and higher utilization of the dc bus voltage than three-phase motors [8, 11, 28, 29, 30]. Bianchi et al [15] demonstrated a fault tolerant five phase low torque 20-slot 18-pole interior rotor permanent magnet machine. This is a unique characteristic of the five phase architecture which is not easily replicated in three phase motors.El-Refaie et al [32] studied 3-, 4-, 5-, and 6-phase high speed motors with fractional slot concentric windings. It could be seen from their study that some of the five-phase slot-pole configuration offered significantly reduced cogging, high winding factor and reduced torque ripple as compared to other configurations.
Axial Flux Motors (AFM):
AFMs can outperform radial flux machines under a set of narrow conditions [18, 19, 20]. AFMs are an attractive solution if the number of poles is high and the axial length is short. Under these conditions, the AFMs can provide both a higher electromagnetic torque and a higher torque density than the RFMs [17].
Most of the hub motors used in LEVs has a very short axial length and relatively large diameter. This arrangement could easily increase torque density and power density by 15% as compared to the radial flux configuration [17]. AFMs also offer balanced motor-stator attractive forces, better heat removal, no rotor back iron and adjustable air gap [33]. Zhang et al. [34] compared the several AFM wheel motors for electric cars, and concluded that the interior PM seemed to be the best compromise in terms of power density, efficiency, compactness, and capability characteristics. Jayasundara et al [10] designed and built a 20-slot/12-pole 5kW five-phase AFM with excellent results.
Winding Methods:
Fractional-slot concentrated-winding (FSCW) PM machines have gained popularity over the last few years. This is mainly due to the several advantages that this type of windings provides. These include high-power density, high efficiency, short end turns, high slot fill factor, low cogging torque, flux-weakening capability, and fault tolerance. In an extensive study [13] involving winding configurations, El-Refaie compared distributed and concentric windings, with respect to the following factors: single- versus double-layer winding layout, fault-tolerance, rotor losses, parasitic effects, interior versus surface PM machines etc.
PM machines used in light electric vehicles are optimized for low speed, high torque application and consequently employ higher pole and slot configuration than high speed PM machines. While using FSCW, rotor losses are of great concern. Higher order harmonics associated with the layout of three-phase motors are known to cause significant rotor losses.
Control Methods:
Several control methods exist today for control of PM machines. Trapezoidal control injects trapezoidal current waveforms into the motor windings. Sinusoidal control injects sinusoidal current. Field oriented control (FOC) converts poly-phase motor currents into a Cartesian coordinate system which rotates with the motor’s synchronous speed. This results in direct control of air gap flux and hence torque. FOC has been known to improve a motor’s dynamic performance substantially. Space vector control is known to increase DC bus voltage utilization by as much as 15% by clever application of the available bus voltage.
Locment et al [35] studied 3-, 5- and 7-phase inverters for low voltage applications. The study stated that the 5- and 7-phase inverters resulted in lower current per phase and leg resulting in higher reliability, better DC bus voltage utilization resulting in more output power, and better utilization of harmonics resulting in more torque. The study showed significant improvement in performance of 5-phase inverters over 3-phase inverters. The performance of 7-phase inverters was marginal over the 5-phase inverters.
In the sub-sections below, we discuss potential customer needs, today’s DDEP technology, technical objectives and technology risks and barriers.
Potential Customer Needs:
Some of the key requirements of these customers are as follows:
Weight: Weight is a significant issue on electric bicycles and light electric vehicles. Portability is important especially in metropolitan cities where an end customer lives in an apartment or uses public transportation system such as rail and bus to get around. Such a customer wants to be able to move her vehicle with ease. Also, many users of these bicycles and vehicles are men and women of age of 40+. Usability is enhanced substantially with portability. Currently, electric propulsion systems weigh as much as 40% of the vehicle weight.
Pedaling: Ability to pedal an electric bicycle is a comforting thought. One does not fear the battery running out of charge. However, one of the issues with the existing propulsion technology is difficulty in pedaling. Most electric bicycles and scooters can not be pedaled easily due to the high cogging torque present in the PM motors. This cogging torque increases significantly as the torque and power of the motor increase. Hence it is critical to reduce the cogging torque of these systems significantly.
Range: Overall efficiency of a propulsion system is quite critical in extending the range of electric bicycles and light electric vehicles. Li-Ion batteries remain inefficient with very high internal impedance. On the other hand, low speed high torque hub motors have been proven to be efficient and quite suitable for light electric vehicles as compared to geared propulsion drives [24]. However, overall propulsion system efficiency remains dismally low. Also, combined efficiency of hub motor and inverter for this application rarely exceeds 73% [37]. Falco and its team have been involved with several DDEP designs in the past for motors ranging from 250 to 1000W [5]. From our experience, the highest efficiency normally occurs between the speeds of 120 to 180 rpm with torque in the range of 8-14N m as shown in Figure 3. This is due to an underlying assumption that a rider spends most of her time in this region. Also, the propulsion system is designed for high torque and a wide speed range. This inherently forces the motor to be extremely inefficient during start-up or high acceleration conditions.
Power: Output power of a propulsion system decides a vehicle’s top speed. In most of Europe, the top speed of electric bicycles is limited to 25km/h and peak power is limited to 250W. However, these limits are deemed inadequate and recent attempts have been made by ETRA (European Twowheel Retailers' Association) to open up these limits further [36]. In Canada, the top speed and power are limited to 32km/h and 500W respectively. In USA, the top speed and power are limited to 32km/h and 750W respectively. In the newest gearless, direct drive propulsion systems, the power density is about 100W/kg. Our target is to increase this power density to about 160W/kg. This would allow producing a 1000W motor for 6kg weight and a 250W motor for about 1.5kg.
Torque: Torque of a propulsion system decides its hill climbing ability. Americans and Europeans on average are bigger. Road and riding conditions are not uniform. A torque of 50-55Nm is needed [24]. Current torque densities are around 7Nm/kg for gearless direct drive propulsion systems. Our target is to increase this torque density to about 11.5Nm/kg. This would allow a 5kg motor to produce 57.5Nm of torque.
Available DDEP Technology for Light Electric Vehicles:
The direct drive propulsion components sold today are low power, inefficient and heavy. These components do not meet existing market needs. The terrains and geographical conditions demand a 500 to 1000W system [24].
Table 1 below summarizes DDEP technology from major manufacturers. Five systems from DK City, Taiwan, Suzhou Bafang, China, BionX, Canada, Heinzmann, Germany and Falco, USA are compared.
Table 2: Comparison with respect to Key Functionality
References:
- Watterson, P.A.; , "An electric assist bicycle drive with automatic continuously variable transmission," Electrical Machines and Systems, 2008. ICEMS 2008. International Conference on , vol., no., pp.2992-2997, 17-20 Oct. 2008
- Chang, L.; , "Comparison of AC drives for electric vehicles-a report on experts' opinion survey," Aerospace and Electronic Systems Magazine, IEEE , vol.9, no.8, pp.7-11, Aug 1994
- Rasmussen, C.B.; Andersen, H.R.; Ritchie, E.; Pedersen, J.K.; , "Design and efficiency comparison of electric motors for low power variable speed drives with focus on permanent magnet motors," Electrical Machines and Drives, 1995. Seventh International Conference on (Conf. Publ. No. 412) , vol., no., pp.428-432, 11-13 Sep 1995
- Chau, K.T.; Chunhua Liu; Jiang, J.Z.; , "Comparison of outer-rotor stator-permanent-magnet brushless motor drives for electric vehicles," Electrical Machines and Systems, 2008. ICEMS 2008. International Conference on , vol., no., pp.2842-2847, 17-20 Oct. 2008
- Dhawan, R.; Soghomonian, Z.; , "Seven phase brush-less synchronous motor with reduced inverter size," Applied Power Electronics Conference and Exposition, 2004. APEC '04. Nineteenth Annual IEEE , vol.2, no., pp. 1099- 1105 vol.2, 2004
- Chan, C.C.; Jiang, J.Z.; Chen, G.H.; Wang, X.Y.; Chau, K.T.; , "A novel polyphase multipole square-wave permanent magnet motor drive for electric vehicles," Industry Applications, IEEE Transactions on , vol.30, no.5, pp.1258-1266, Sep/Oct 1994
- Waikar, S.; Gopalarathnam, T.; Toliyat, H.A.; Moreira, J.C.; , "Evaluation of multiphase brushless permanent magnet (BPM) motors using finite element method (FEM) and experiments," Applied Power Electronics Conference and Exposition, 1999. APEC '99. Fourteenth Annual , vol.1, no., pp.396-402 vol.1, 14-18 Mar 1999
- Jing Huang; Corzine, K.A.; Hutson, C.M.; Shuai Lu; , "Extending voltage range and reducing torque ripple of five-phase motor drives with added voltage harmonics," Applied Power Electronics Conference and Exposition, 2008. APEC 2008. Twenty-Third Annual IEEE , vol., no., pp.866-872, 24-28 Feb. 2008
- Parsa, L.; Toliyat, H. A.; Goodarzi, A.; , "Five-Phase Interior Permanent-Magnet Motors With Low Torque Pulsation," Industry Applications, IEEE Transactions on , vol.43, no.1, pp.40-46, Jan.-feb. 2007 doi: 10.1109/TIA.2006.887235
- Jayasundara, J.W.K.K.; Munindradasa, D.A.I.; , "Design of Multi Phase In-Wheel Axial Flux Permanent Magnet Motor for Electric Vehicles," Industrial and Information Systems, First International Conference on , vol., no., pp.510-512, 8-11 Aug. 2006
- Parsa, L.; Goodarzi, A.; Toliyat, H.A.; , "Five-phase interior permanent magnet motor for hybrid electric vehicle application," Vehicle Power and Propulsion, 2005 IEEE Conference , vol., no., pp. 631- 637, 7-9 Sept. 2005
- Munoz, A.R.; Liang, F.; Degner, M.W.; , "Evaluation of Interior PM and Surface PM Synchronous machines with distributed and concentrated windings," Industrial Electronics, 2008. IECON 2008. 34th Annual Conference of IEEE , vol., no., pp.1189-1193, 10-13 Nov. 2008 doi: 10.1109/IECON.2008.4758123
- L-Refaie, A.M.; , "Fractional-Slot Concentrated-Windings Synchronous Permanent Magnet Machines: Opportunities and Challenges," Industrial Electronics, IEEE Transactions on , vol.57, no.1, pp.107-121, Jan. 2010 doi: 10.1109/TIE.2009.2030211
- Shah, M.R.; EL-Refaie, A.M.; , "Eddy-Current Loss Minimization in Conducting Sleeves of Surface PM Machine Rotors With Fractional-Slot Concentrated Armature Windings by Optimal Axial Segmentation and Copper Cladding," Industry Applications, IEEE Transactions on , vol.45, no.2, pp.720-728, March-april 2009
- El-Refaie, A.M.; Jahns, T.M.; , "Impact of Winding Layer Number and Magnet Type on Synchronous Surface PM Machines Designed for Wide Constant-Power Speed Range Operation," Energy Conversion, IEEE Transactions on , vol.23, no.1, pp.53-60, March 2008
- Shah, M.R.; El-Refaie, A.M.; , "End effects in multi-phase fractional-slot concentrated-winding surface Permanent Magnet synchronous machines," Energy Conversion Congress and Exposition, 2009. ECCE 2009. IEEE , vol., no., pp.3798-3805, 20-24 Sept. 2009
- Cavagnino, A.; Lazzari, M.; Profumo, F.; Tenconi, A.; , "A comparison between the axial flux and the radial flux structures for PM synchronous motors," Industry Applications, IEEE Transactions on , vol.38, no.6, pp. 1517- 1524, Nov/Dec 2002
- Parviainen, A.; Niemela, M.; Pyrhonen, J.; Mantere, J.; , "Performance comparison between low-speed axial-flux and radial-flux permanent-magnet machines including mechanical constraints," Electric Machines and Drives, 2005 IEEE International Conference on , vol., no., pp.1695-1702, 15-15 May 2005
- Ronghai Qu; Aydin, M.; Lipo, T.A.; , "Performance comparison of dual-rotor radial-flux and axial-flux permanent-magnet BLDC machines," Electric Machines and Drives Conference, 2003. IEMDC'03. IEEE International , vol.3, no., pp. 1948- 1954 vol.3, 1-4 June 2003
- Rahman, Z.; , "Evaluating radial, axial and transverse flux topologies for 'in-wheel' motor," Power Electronics in Transportation, 2004 , vol., no., pp. 75- 81, 21-22 Oct. 2004
- Markovic, M.; Perriard, Y.; , "Optimization Design of a Segmented Halbach Permanent-Magnet Motor Using an Analytical Model," Magnetics, IEEE Transactions on , vol.45, no.7, pp.2955-2960, July 2009
- Wei Min; Ming Zhang; Yu Zhu; Badong Chen; Guanghong Duan; Jinchun Hu; Wensheng Yin; , "Analysis and Optimization of a New 2-D Magnet Array for Planar Motor," Magnetics, IEEE Transactions on , vol.46, no.5, pp.1167-1171, May 2010
- Jones, W.D.; , "Putting Electricity Where The Rubber Meets the Road [NEWS]," Spectrum, IEEE , vol.44, no.7, pp.18-20, July 2007
- Muetze, A.; Ying Chin Tan; , "Modeling and Analysis of the Technical Performance of DC-Motor Electric Bicycle Drives Based on Bicycle Road Test Data," Electric Machines & Drives Conference, 2007. IEMDC '07. IEEE International , vol.2, no., pp.1574-1581, 3-5 May 2007
- van Schalkwyk, D.J.; Kamper, M.J.; , "Effect of Hub Motor Mass on Stability and Comfort of Electric Vehicles," Vehicle Power and Propulsion Conference, 2006. VPPC '06. IEEE , vol., no., pp.1-6, 6-8 Sept. 2006
- Yong Chen; Zhongkui Lu; , "Simulation analysis of vertical and lateral dynamics of an electric vehicle driven by two rear hub-motors," Mechatronics and Automation, 2009. ICMA 2009. International Conference on , vol., no., pp.3613-3618, 9-12 Aug. 2009
- Kazmin, E.V.; Lomonova, E.A.; Paulides, J.J.H.; , "Brushless traction PM machines using commercial drive technology, Part I: Design methodology and motor design," Electrical Machines and Systems, 2008. ICEMS 2008. International Conference on , vol., no., pp.3801-3809, 17-20 Oct. 2008
- Levi, E.; Jones, M.; Vukosavic, S.N.; Toliyat, H.A.; , "Steady-State Modeling of Series-Connected Five-Phase and Six-Phase Two-Motor Drives," Industry Applications, IEEE Transactions on , vol.44, no.5, pp.1559-1568, Sept.-oct. 2008 doi: 10.1109/TIA.2008.2002268
- Parsa, L.; Toliyat, H.A.; , "Five-phase permanent-magnet motor drives," Industry Applications, IEEE Transactions on , vol.41, no.1, pp. 30- 37, Jan.-Feb. 2005
- Parsa, L.; Toliyat, H.A.; , "Fault-tolerant five-phase permanent magnet motor drives," Industry Applications Conference, 2004. 39th IAS Annual Meeting. Conference Record of the 2004 IEEE , vol.2, no., pp. 1048- 1054 vol.2, 3-7 Oct. 2004
- Bianchi, N.; Bolognani, S.; Dai Pre, M.; Fornasiero, E.; , "Post-fault operations of five-phase motor using a full-bridge inverter," Power Electronics Specialists Conference, 2008. PESC 2008. IEEE , vol., no., pp.2528-2534, 15-19 June 2008
- El-Refaie, A.M.; Shah, M.R.; Ronghai Qu; Kern, J.M.; , "Effect of Number of Phases on Losses in Conducting Sleeves of High Speed Surface PM Machine Rotors," Industry Applications Conference, 2007. 42nd IAS Annual Meeting. Conference Record of the 2007 IEEE , vol., no., pp.1522-1529, 23-27 Sept. 2007
- D. C. Hanselman, Brushless Permanent-Magnet Motor Design. New York: McGraw-Hill, 1994.
- Z. Zhang, F. Profumo, and A. Tenconi, “Axial flux machine for electric vehicles,” Elec. Mach. Power Syst., vol. 24, pp. 883–896, 1996.
- Locment, F.; Bruyere, A.; Semail, E.; Kestelyn, X.; Bouscayrol, A.; Dubus, J.M.; , "Comparison of 3-, 5- and 7-leg Voltage Source Inverters for low voltage applications," Electric Machines & Drives Conference, 2007. IEMDC '07. IEEE International , vol.2, no., pp.1234-1239, 3-5 May 2007
- Over 40 companies registered for ETRA-meeting on European regulations for electric bicycles at Eurobike - http://www.etra-eu.com/newsitem.asp?type=3&id=3699305
- Lomonova, E.A.; Vandenput, A.J.A.; Rubacek, J.; d'Herripon, B.; Roovers, G.; , "Development of an improved electrically assisted bicycle," Industry Applications Conference, 2002. 37th IAS Annual Meeting. Conference Record of the , vol.1, no., pp. 384- 389 vol.1, 2002
- http://www.bionx.ca/en/products/how-to-choose/
- Letelier, A.; Tapia, J.A.; Wallace, R.; Valenzuela, A.; , "Cogging torque reduction in an axial flux PM machine with extended speed range," Electric Machines and Drives, 2005 IEEE International Conference on , vol., no., pp.1261-1267, 15-15 May 2005
- http://www.pikeresearch.com/research/electric-two-wheel-vehicles
- Colker, D.; “Consumer Watch; Electricity boosts leg power for bicyclists; Hybrid bikes that use battery and muscle power have come a long way. Fold-up models that can be stowed at the office also see improvements” - Los Angeles Times, Sep, 2008